Understanding Flash in Compression Molding
Compression molding is a manufacturing technique used to shape composite materials like SMC (sheet molding compound) and BMC (bulk molding compound) into intricate parts.The process involves loading the material into a heated mold cavity, which is closed under pressure to force the material into every corner and crevice of the mold.One thing that often happens during this process is the formation of “flash” — excess material that squeezes out of the mold. At first, you might think of flash as a nuisance, but it’s actually really important for the success of the molding operation.This article will go into more detail about why flash is a must in compression molding and how it improves the quality and efficiency of the production process.
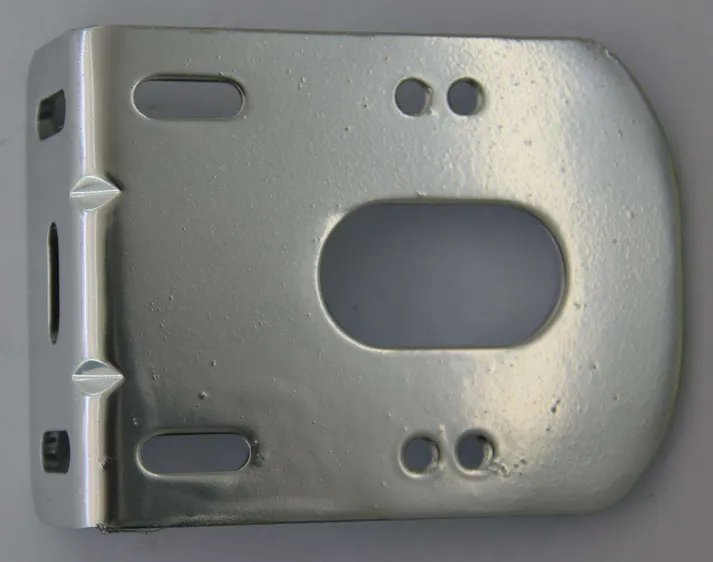
Importance of Flash in Compression Molding
1. Making Sure the Mold is Full
Flash is like your trusty indicator that the mold is full.In compression molding, you’ve got to apply pressure to force the material into every nook and cranny of the mold. Flash is like your little helper, helping to balance out any differences in how the material is distributed. It makes sure that even the tiny details of the mold are spot on when you’re done.
2. Improving Part Integrity
By keeping pressure consistent in the mold, flash helps make the part stronger. It lets some of the extra material out, which helps avoid problems like voids, weak spots, or weld lines that could make the part weaker. This makes sure the final product meets the high quality standards needed for different uses.
3. Compensating for Material Variability
SMC and BMC composite materials can have slight variations in properties like viscosity, density, and flow characteristics. Flash helps deal with these inconsistencies by allowing excess material to be moved out of the mold cavity. This ensures that the part is consistently filled and free from defects caused by uneven material flow.
4. Protecting the Mold
Flash acts as a protective layer between the mold surfaces and the material being molded.The high pressures involved in compression molding can potentially cause damage to the mold surfaces or lead to uneven wear.By allowing some material to escape as flash, the mold is subjected to less stress, which helps prolong its lifespan and reduce maintenance costs.
5. Supporting Demolding Processes
Flash can make the demolding process easier and more efficient by providing extra gripping points for ejector pins or trimming tools, which is really useful when dealing with parts that have complex shapes or materials that are prone to sticking to the mold cavity. This helps reduce production time and costs.
But flash can also cause problems.
While flash is a key part of the compression molding process, there are some challenges that manufacturers need to deal with:
- Material Waste: Flash is material that isn’t used in the final product, which increases costs.But advances in recycling technology have made it possible to recover and reuse flash material in some cases, reducing waste.
- Secondary Operations: Trimming flash from the molded part requires extra steps in the production process, which can increase labor and equipment costs.Efficient trimming methods and automated systems are being developed to streamline this process and reduce costs.
- Mold Design Complexity: Designing molds that can handle controlled flash formation requires a lot of expertise in mold design and engineering. This adds complexity to the overall manufacturing process, but with proper planning and execution, it can be managed effectively.
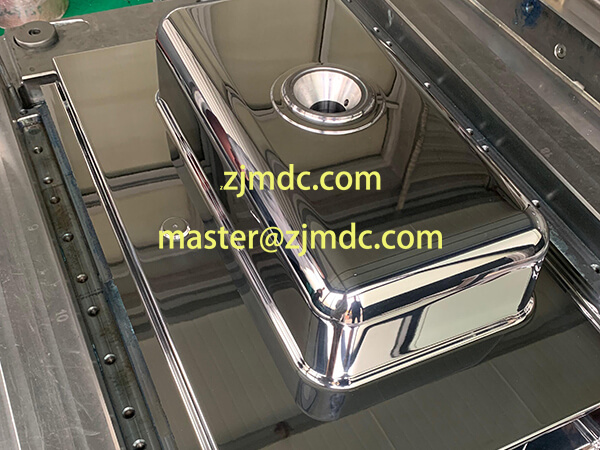
Minimizing excessive flash is key.
Flash is necessary for the success of the compression molding process, but too much can lead to inefficiencies and increased costs.Here are some strategies for managing flash effectively:
- Optimized Mold Design: Molds that are designed with precision and tight tolerances can help minimize flash while making sure the mold cavity is fully and uniformly filled.Using advanced mold design techniques and materials can also help improve the efficiency of the molding process.
- Accurate Material Loading: Loading the mold with the right amount of material can help reduce excess flash and improve product consistency. This means measuring and controlling the material feeding process accurately.
- Proper Mold Maintenance: You should regularly check and clean the mold parting lines to prevent excessive flash formation due to mold wear or damage. This includes inspecting the mold for any signs of wear or damage and making necessary repairs or replacements.
- Automated Trimming: Automated trimming systems can really help with this. They can automate the process of removing flash from molded parts, which can reduce labor costs, improve consistency, and increase production efficiency.
Conclusion
Flash is a key part of the compression molding process. It’s essential for filling the mold completely, making the parts stronger, and protecting the mold from wear and tear. Even though it can lead to material waste and extra steps, the pros of flash far outweigh the cons. By getting a handle on how flash works in the molding process and having good strategies for dealing with it, manufacturers can make top-notch parts without wasting materials or spending more money. And with more and more innovation in mold design, material handling, and automation technologies, the compression molding process is only going to get better and better.